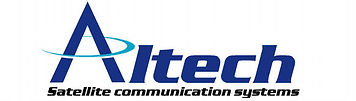
Propagation of V-band (50 – 75 GHz) and W-band (75 – 110 GHz) electromagnetic waves through the troposphere are fraught with many challenges. The data needed to fully characterize the channel for these bands is still being collected; and current physical models are mostly based on extrapolations from lower frequencies. The Italian Space Agency (ASI) appears to be conducting the most aggressive collection of propagation data for these bands.
Electromagnetic waves are subject to many propagation impairments. The physical processes affecting key wave parameters are [1]:
a) Amplitude: absorption, scattering, refraction, diffraction, multipath resulting in fading and scintillation.
b) Phase: scattering, refraction, diffraction, and multipath.
c) Polarization: depolarization by: absorption, scattering, refraction and multipath
d) Frequency: dispersion.
e) Bandwidth: scattering, refraction, diffraction, multipath, and dispersion.
f) Angle-of-Arrival: scattering, refraction, diffraction and multipath.
Relating the above physical processes (absorption, scattering, refraction, diffraction, multipath, dispersion) to the meteorology of the troposphere, and then back to electromagnetic wave propagation, is the challenge.
At the V/W-band frequencies the principal propagation impairments (in approximately decreasing order of effect) are:
a) Rain Attenuation: mainly by scattering, but also absorption.
b) Rain Depolarization: due to rain drop geometry.
c) Gaseous Attenuation: molecular absorption.
d) Cloud and Fog Attenuation: by both water droplets and ice.
e) Ice Depolarization: ice crystals (both plate and needle-shaped) in clouds.
f) Tropospheric Scintillation: variation in atmospheric refractive index.
g) Radio Noise: re-emissions electromagnetic energy by molecules in the atmosphere.
The importance of some of these impairments to Q/V-band communication has been realized only recently [2]. To illustrate the proposed approach to WSCE, let’s briefly recount issues of: Cloud Attenuation, Rain Depolarization and Ice Depolarization.
Cloud attenuation was initially disregarded in satellite communications. Now however 1 – 2 dB attenuation at frequencies of 30 GHz with elevation angles of 30 degrees is recognized as a common occurrence (in the mid latitudes). Characterization of this impairment will require cataloguing clouds by type, water density and altitude.
Rain depolarization also took time to appreciate[3]: “The significance of depolarization due to the propagation medium was not realized until more than a dozen years after geostationary satellites were in commercial service.” It is now understood that rain depolarization is caused by two separate means: differential attenuation and differential phase effects – both between the major and minor axes of ellipsoidal raindrops. It is also understood that differential phase effects dominate at frequencies below 10 GHz and differential attenuation effects dominate at frequencies above 20 GHz. This seems to be in accordance with theory, so our experiment plan will focus on the latter.
Ice depolarization (as opposed to rain depolarization) is caused primarily by differential phase shift [1]. The ice crystals are aligned along electrostatic fields within the clouds, but they oscillate along their axes so there is less coherence to their alignment than that of rain. Initial quantitative measures were started in the early 1980’s. Studies attempting to correlate with ice depolarization with path attenuation, elevation angle and scintillation have shown mixed results. It is now believed that stratus rainfall is always accompanied by ice depolarization; and that changes should be made to rain rate models that factor in the yearly proportion of stratus rainfall.
Cloud Attenuation, Rain Depolarization and Ice Depolarization are propagation effects that were discovered only as higher frequency bands were examined. Their relevant issues are recounted to emphasize three points:
a) Lack of a complete empirical foundation for physical channel models;
b) Lack of data on interrelationships between phenomena (e.g., depolarization and scintillation);
c) Necessity of a complete meteorological instrumentation package for this effort.
These points are addressed in turn below.
Well-known current propagation impairment models are [4]:
P.372-10 Radio noise
P.676-9 Attenuation by atmospheric gases
P.834-6 Effects of tropospheric refraction on radiowave propagation
P.837 Characteristics of precipitation for propagation modelling
P.838 Specific attenuation model for rain for use in prediction methods
P.839 Rain height model for prediction methods
P.840 Attenuation due to clouds and fog
P.1057-2 Probability distributions relevant to radio propagation modelling
P.1322 Radiometric estimation of atmospheric attenuation
P.1623 Prediction method of fade dynamics on Earth-space paths
However current ITU rain attenuation models are mostly based on empirical observations from frequencies below 20 GHz. Extrapolation of the ITU and other models to V/W bands results in a wide variation of attenuation predictions [5 ]. Several of the other ITU models are also based on extrapolation to higher frequencies. As seen by the example of ice depolarization above, this may not always result in the best answer.
The WSCE clearly attempts to redress this issue, but the three examples above demonstrate that a full instrumentation suite is warranted because as new frequency bands are explored, new phenomena have been encountered.
To illustrate, although most measurement campaigns measure only cross-polarized interference (XPI), ours will measure cross-polarized discrimination (XPD). The OLYMPUS satellite measured XPD by using two beacons in quick succession: one horizontally polarized, the other vertically polarized. This was done for the 4 – 12 GHz range. This proved useful for correlating scintillation with depolarization. As noted in [3] “Turbulent scintillation in a relatively clear air in the vertical polarization orientation was statistically larger than in the horizontal polarization orientation, while the reverse was the case in humid tropospheric scintillation in light rain conditions.”
The lack of data on interrelationships between phenomena is alluded to in reports on the DAVID (Data and Video Interactive Distribution) experiment conducted by ASI (De Fina, Ruggieri and Vittoria Bosisio 2003). They have decided not to use the ITU models for combining the attenuations due to rain, scintillation, clouds, oxygen and water vapor. Their specific formula for total system power loss due to these attenuations is shown in Eq. 1:
3.1 Physical Channel Environment


Showing contributions due to attenuation by rain, scintillation, clouds, oxygen and water vapor when a system outage probability and elevation angle are given by Eq. 2:
This is significant in DAVID (and possibly in WSCE) as this is one means of predicting link margins for fade mitigation techniques. It also highlights the importance of correlating these different propagation impairments in a satisfactory manner, particularly in exercising fade mitigation techniques (FMT).
The necessity for a complete meteorological instrumentation package for this effort may seem obvious, but it is related to the first two points. Experiments with the ATS-6 satellite confirmed ideas about cant angles for ensembles of rain drops in storms [1]. However when anaomalous depolarization was detected, it was only suspected that either dry snow or ice crystals were the cause. It was not until range-gated radars were used to sample the slant path at the same time as the satellite beacon that this could definitively be ascribed to ice crystals.
Therefore the Experiment Plan will include an assortment of radars, multi-spectral radiometers [6], infrared sensors, and sensitive meteorological equipment. The use of now casting (obtaining current weather data and imagery to predict conditions a short time in advance) will be explored.
As rough guidelines for the hardware section of the proposal, summary results are available from a few published reports to augment the figures given in the solicitation.
Preliminary W-band results are available from ten years of radiosoundings collected in non-rain conditions, twice a day for ten years [7]. These results could provide some rough preliminary guidelines for link margins.
The effects of water vapor, clouds and oxygen were measured and modelled. For a non-precipitating atmosphere attenuation (relative to other frequencies) at a 30 degree slant path is: 7 dB at 72 GHz and 10 dB at 93 GHz for 1% of the time. This will probably require compensation with an increased static margin (e.g. more power or antenna gain). Antenna noise level at 1% exceedance varied from about 175 K to 300 K.
Rain attenuation is significant. Even if site diversity is used to achieve a 60% to 70% gain, an attenuation of 30 dB at 10E-4 and 10 dB at 0.1% of the time can be expected. This may imply a low availability system without offsetting gains from error coding or increased antenna EIRP.
Depolarization due to the non-spherical shape of water drops and ice crystals is significant; but can also be modelled. However, angle of arrival fluctuations and scintillation do not conform well to extrapolated standard ITU models and need further study.
The W-Band Analysis and Verification (WAVE) study also has some initial total attenuation estimates [8] done by simulation. At 1% of the time with elevation angles ranging from 45 – 90 degrees at 94 GHz, the total attenuation varies from 11.69 to 14.92 dB.
Finally, an economical way of enhancing the data collection effort for WCSE would be to incorporate the resulting data set into the “Global Archive of Propagation Measurements for SatCom Systems” [9]. The European Space Agency (ESA) is sponsoring this effort: “to gather and archive experimental data collected during satellite propagation experiments” [9]. It will include the following past experiments that may be helpful:
a) OLYMPUS: 12.5, 19.8 and 29.7 GHz beacons;
b) ITALSAT: 18.7, 39.6 and 49.5 GHz beacons;
c) ACTS: 20.2 and 27.5 GHz beacons.
If this is pursued, the results of WAVE and the DAVID experiment, both by ASI, will be asked for.
In summary, the Experiment Plan for WSCE should:
a) Be circumscribed by known and well-understood theory (e.g., rain depolarization will focus on differential attenuation and discount differential phase effects).
b) Be open to the possibility that new phenomena may be unveiled (e.g., the near-permanent presence of statistically aligned ice crystals in stratus clouds).
c) Open to the possibility that the relations between observed phenomena are not easily correlated; and that the current ITU models are only a starting point.
d) Include as full a suite of meteorological and communications instrumentation as possible.
e) If possible, coordinate the collected data with ESA and other agencies to expand understanding and facilitate physical model training and development.
Acronyms:
ASI Italian Space Agency
BER Bit Error Rate
DAVID Data and Video Interactive Distribution
ESA European Space Agency
FMT Fade Mitigation Technique
ITU International Telecommunications Union
ITU-R ITU Radiocommunication
WSCE W/V-band Satellite Communications Experiment
WAVE W-band Analysis and Verification
XPD Cross-Polarized Discrimination
XPI Cross-Polarized Interference
References
[1] Ippolito Jr., Louis. Satellite Communications Systems Engineering: Atmospheric Effects, Satellite Link Design and System Performance. Chichester, West Sussex: John Wiley & Sons, 2008.
[2 ]Pratt, Timothy, Charles Bostian, and Jeremy Allnutt. Satellite Communications. Hoboken, NJ: John Wiley & Sons, 2003.
[3]Allnutt, J.E. Satellite-to-Ground Radiowave Propagation. Six Hills Way, Stevenage: The Institution of Engineering and Technology, 2011.
[4]ITU. Radiowave propagation. 2012 йил 16-October. http://www.itu.int/rec/R-REC-P/en (accessed 2012 йил 26-November).
[5]Brost, G. A., and W. G. Cook. “Analysis of Empirical Rain Attenuation Models for Satellite Communications at Q and W Band.” 6th
European Conference on Antennas and Propagation (EUCAP). Prague: IEEE, 2012. 1455-1459.
[6]Amaya, Cesar, and Pierre Bouchard. “Analysis of Fade Dynamics and Cloud Absorption using Beacon Data and Atmospheric Profiles.” EuCAP 2009. Berlin: IEEE, 2009. 3616-3620.
[7]Capsoni, C., L. Luini, R. Nebuloni, A. Paraboni, and C. Riva. “Electromagnetic Propagation at Frequencies above 50 GHz: the Challenge of the Atmosphere.” Antennas and Propagation, 2009. EuCAP 2009. 3rd European Conference on. IEEE, 2009. 2668 - 2672.
[8]Jebril, A., M. Lucente, T. Rossi, M. Ruggieri, and S. Morosi. “New Perspectives in the WAVE W-Band Satellite Project.” Boston: Springer-Verlag, 2008. 717-725.
[9]Lemorton, J., L. Castanet, G. Blarzino, and G. Carrie. "Global archive of propagation measurements for satellite communication systems." 3rd European Conference on Antennas & Propagation, 2009: 3623-3627.